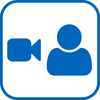
Video-Forschungsbeiträge
2021
008 | Load-specific variant generation of bead cross sections in sheet metal components by unidirectional carbon fibre reinforcement
Michael Ott, Patrick Haberkern, Maximilian Gruber, Christoph Hartmann, Theodora Risch, Cristoph Wunderling, Albert Albers, Wolfram Volk
Virtual IDDRG - Stuttgart - June 21st - July 2nd 2021
Beads are widely used to stiffen sheet metal components subjected to bending loads. Often, these bead-stiffened parts are used in product variants that differ significantly in the amount of acting loads. Lamination of unidirectional carbon fibre reinforced plastic (UD-CFRP) on the top flange area of individual beads represents a method for further increasing weight-specific stiffness: By varying the number of plies, a specifically configured component is obtained for each of the load cases. As a result, no changes to the forming tools are necessary and a minimum amount of the UD-CFRP material is required. In this work, a complete manufacturing process for a fibre reinforced bead was developed: First, a bead cross section geometry with an adapted top flange area to accommodate the UD-CFRP plies was designed and stamped into pre-stretched sheet samples of DX56 steel. Subsequently, the suitability of several surface pre-treatment processes to achieve sufficient bond strength of the composite bead was experimentally investigated and the UD-CFRP plies were applied by lamination. Final bending tests quantified the achievable stiffening effect of the investigated bead variants, showing a significant increase of the maximum supportable load compared to the standard non-reinforced cross-section.
007 | Potentials for material card validation using an innovative tool
Matthias Eder, Maximilian Gruber, Niko Manopulo, Wolfram Volk
For the numerical description of the material behavior of sheet metals, there is a large diversity of models. These models vary strongly in terms of complexity and calibration effort. Before using a material model for numerical analyses, it is essential to validate it. The paper presents an innovative tool that allows generating a well-founded database for a comprehensive validation of the yield locus description with few and simple tests: the MUC-Test (Material Under Control). The special geometry of the punch and die generates complex strain distributions in sheet metal samples during forming. The experiment covers the strain range between uniaxial and equibiaxial tension. The contribution shows the potential of this innovative tool by using the test results of three different material classes. Using numerical analyses, sensitivities of different material model parameters were investigated. Thereby, the focus lies on the curvature of the yield locus in the area between uniaxial and equibiaxial stress state.
006 | Parameterized data handling for forming tool tryout
The forming tool design process generates large amounts of data up to the first falling parts. On the one hand, simulation results, geometric measurements and design models originate from different software tools, which leads to a non-consolidated set of data inventory. On the other hand, the total data volume is hard to handle economically. A stable and user-friendly data structure for overarching tool tryout is missing. Often several experience-based iterations are necessary to derive the tool's working surfaces, which is both time- and resource consuming and even may lead to postponed start of production. Meanwhile, early-generated data does not involve into the manual optimization process. Therefore, in this paper a parameterized data handling methodology is introduced, which enables systematic reverse engineering, data consolidation, and springback compensation. Each generated dataset during tryout is traced back to a mathematical description of geometry using so called control points (B-Spline model). Through, the parameterized description, the different data formats interact straightforward and need minimum storage. The developed concept is demonstrated for the springback analysis of a forming component using design models and numerical data.
Springback is a fundamental challenge in sheet metal forming and a critical issue with respect to part quality and dimensional accuracy. To overcome the problem, different strategies for springback compensation have emerged in recent years. In this paper, we give a review on fundamental strategies of compensation for deviations in sheet metal forming and embed them, for the first time, in a general description framework rooted in the solution of ill-posed problems. On this basis, we conclude by briefly comparing different approaches and elaborate on future challenges and chances that naturally arise from the presented problem formulation.
2020
004 | Influence of non-proportional load paths and change in loading direction on the failure mode of sheet metals
W. Volk, R. Norz, M. Eder, H. Hoffmann
CIRP Annals, Volume 69, Issue 1, 2020, Pages 273-276
Many studies have shown that failure following non-proportional load paths cannot be predicted by a linear Forming Limit Curve (FLC), as the deformation history and a change in loading direction influence the formability and failure mode. In this paper, the different failure modes due to different load paths are investigated, for the first time, by conducting Nakajima tests with pre-formed specimens. The main objective of the investigation is to better understand the influence of pre-forming and change in loading direction on the formability. To predict this behaviour, regardless of the failure mode, the Generalized Forming Limit Concept (GFLC) is extended.
003 | Prediction of limit strains during non-proportional load paths with a change in loading direction
IOP Conference Series: Materials Science and Engineering, Volume 967, International Deep-Drawing Research Group (IDDRG 2020) 26-30 October 2020, Seoul, South Korea
Many different models have been published to predict failure after non-proportional load paths. Most of those models are phenomenological and heuristical models. They require a profound knowledge about the material. Examples are the enhanced Modified Maximum Force Criterion (eMMFC), the Polar Effective Plastic Strain-model (PEPS) or the Generalized Forming Limit Concept (GFLC). In addition to the load path, the loading direction has a significant influence on the formability of sheet metals. The mentioned models currently neglect this influence. By extending the GFLC-model by the parameter of loading direction, this influence is taken into account. By analyzing an acceptable number of bi-linear experiments, it is possible to calibrate the proposed model for a micro-alloyed steel HC340LA. Therewith an arbitrary load path with a change in loading direction can be evaluated. The results of this contribution show the effectiveness of this approach by different experiments.
002 | Development of inline closed-loop vibration control in progressive die stamping using finite element simulation
F. Steinlehner, M. Ott, D. Budnick, A. Weinschenk, S. Laumann, M. Worswick, W. Volk
IOP Conference Series: Materials Science and Engineering, Volume 967, International Deep-Drawing Research Group (IDDRG 2020) 26-30 October 2020, Seoul, South Korea
Modern progressive dies are increasingly equipped with integrated sensors and actuators, thus enabling an improvement of the manufacturing process and an inline monitoring of the component quality. The design of the tooling is determined at an early stage and is largely based on the desired product and the specific production stages such as blanking, forming and punching. Nevertheless, malfunctions such as strip vibrations cannot always be foreseen and may occur during operation time, which may lead to reduced component quality and in the worst case to component collision or a damage of the tooling. Therefore, complex reworking or a reduction of the stroke rate and thus lower output quantity are often necessary for a reliable and stable process. Strip vibrations are often caused by the highly dynamic transportation of the strip in the tool and can have various causes. Among others, passive tooling components such as spring-loaded, hard stop limitedstrip liftercan be the cause of such vibrations. Strip lifterare always necessary when three-dimensional components are produced and have to be lifted out of the die for the feeding phase. The feeding phase takes place between two strokes of the stamping process. This work is aiming for a control strategy to suppress strip vibrations in various progressive die stamping processes based on closed-loopcontrolled active strip lifter. These strip liftercombine the spring-loaded passive standard striplifter with an additional PID-controlled actuator. Taking the dynamics of the flexible strip during operation into account, a Finite Element Analysis (FEA) model of a progressive die tooling system is created. For the design of the control algorithm, the FEA model is connected to an environment for model-based design in a co-simulation. This approach allows modelling the influence of arbitrary control parameter settings on the movement curve of the strip, aiming for an increased stroke rate.
Many industrial sheet metal parts experience a non – proportional loading history. The determination of such non – proportional loading paths on a laboratory scale is still very challenging and time - consuming. Most tests require different machines, tools and measurement techniques. Norz and Volk [1] have presented a new approach to create arbitrary non – proportional loading paths by using a cruciform specimen in combination with a draw bead tool. This simple experimental setup lead to a significant reduction in the experimental effort. It allows the generation of arbitrary loading paths by using only one machine, one tool and one specimen geometry without any further processing of the specimen between the forming steps. Nevertheless, the strain path could only be controlled manually after a certain forming step. In this paper, an in-line strain measurement was used to observe the real – time strain path during the experiment. This information was used to create a semi-automatic approach in which the draw bead height is adapted in order to create a prescribed strain path.